In part one of our heat performance test series, we learn how Silcolloy® performs under extreme temperature. In part 2 we'll learn how to improve Silcolloy's thermal performance!
What Happens When Silicon is Heated?
One of the key advantages of SilcoTek’s CVD coatings over polymer-based coatings is their ability to be used for high temperature applications. Among our current commercially available coatings, Silcolloy® (hydrogenated amorphous silicon) has been the recommendation for most high temperature applications (≥ 450°C).
In this blog post you will learn:
- How to test and evaluate the thermal performance of silicon coatings.
- How Silcolloy® performs under extreme heat.
- How hydrogenated amorphous silicon changes when exposed to high temperature.
|
Amorphous silicon (a-Si) transitions to crystalline silicon (c-Si) at around 700°C as part of its intrinsic physical properties, but when in direct contact with a metal substrate, crystallization of amorphous silicon can be catalyzed by the metal and occur at a lower temperature. The degree of which depends on the type of metal. Crystallization causes coating degradation and facilitates outward metal diffusion, which undermines the coating’s barrier properties. In this blog we'll discuss how silicon coating (Silcolloy) properties change when exposed to extreme heat. In a follow-up post, we'll discuss how a change to the coating application process improved the thermal performance of Silcolloy.

Background:
High temperature thermal stability is one of the key advantages of SilcoTek’s silicon-based CVD coatings when compared to traditional polymer-based organic coatings. Unlike organic coatings that soften, decompose or melt, usually in the 200-300°C temperature range, SilcoTek’s coatings can handle at least 400-500°C. The specific maximum temperature depends on coating type, for coatings that have a surface functionalization chemistry (such as SilcoNert® 2000 and Dursan®) the maximum temperature ranges from 400-500°C, for coatings that are comprised of one bulk layer with no surface functionalization chemistry (such as Silcolloy® and Dursox®) the maximum temperature increases to 700°C.
In the case of coatings that come with surface functionalization, the functionalization (i.e. the uppermost layer of the coating that is too thin to be measured) starts to decompose in the 400-500°C range, leading to gradual loss of properties such as ultra-inertness and hydrophobicity, yet the bulk of the coating still remains to provide basic barrier protection to the underneath substrate.

Among the current commercially available SilcoTek® coatings, Silcolloy has been the go-to recommendation for most high temperature (≥ 450°C) applications. Silcolloy is made purely of hydrogenated amorphous silicon (a-Si) with no additional surface functionalization. As amorphous silicon is an excellent barrier against corrosion, especially oxidation (which is a form of corrosion), Silcolloy seems to have the best chemistry (among SilcoTek products) to handle high temperature applications.
However, Silcolloy does have its Achilles’ heel in that amorphous silicon starts to crystallize at around 700°C. This is an intrinsic materials property of amorphous silicon (a-Si), as shown in our previous TGA-DSC study on a-Si particles. The TGA-DSC experiment investigated stand-alone amorphous silicon particles (not a coating attached to a substrate), therefore the properties measured reflect the inherent attribute of amorphous silicon regardless of substrate. When amorphous silicon is deposited as a coating on top of a metal substrate, the silicon crystallization may often occur below 700°C. Because many metals catalyze the silicon coating, the crystallization process is triggered before the coating reaches the intrinsic crystallization temperature. For example, a-Si deposited on aluminum starts to crystallize as early as around 400°C, while for stainless-steel, this transition occurs around 600°C.
Once crystallized, silicon becomes much more susceptible to oxidation, and the transformation from amorphous to crystalline may lead to coating degradation. Therefore, it is important to eliminate the occurrence of such premature crystallization caused by metal, to maximize the potential of a-Si within its material limit. This blog discusses the a-Si transition, our second blog post in our series will discuss SilcoTek’s recent improvements on this front to deliver a more thermally stable Silcolloy coating.
Testing and Results
Figure 1 records the visual evolution of the Silcolloy coating starting at the baseline condition (as-deposited), and going through the different thermal exposures (all samples were held at the peak temperature for six hours in air). The standard operating procedure (SOP) coating showed a more drastic change in color once reaching 700°C, turning dark brown first, then completely black at 800°C
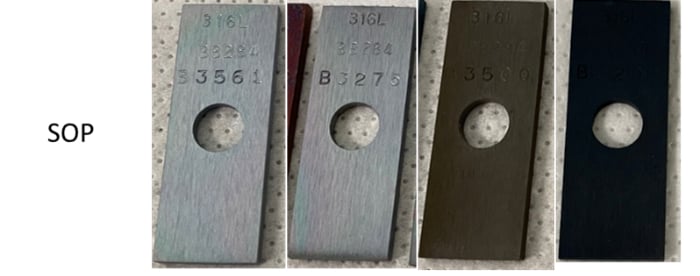
Figure 1: Visual evolution of Silcolloy treated 316L going through thermal exposure (carried out for 6 hours in air)
SEM (Scanning Electron Microscope) images were taken on individual coupons through the thermal exposure stages, to correlate the macroscopic visual evolution with microscopic structural changes in the coating, as shown in Figure 2.
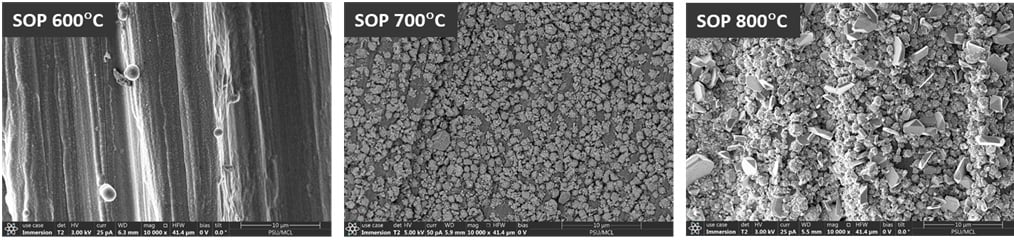
Figure 2: SEM images of SOP Silcolloy (taken at 10K magnification) after thermal exposure to 600, 700 and 800°C for 6 hours in air
EDS (Energy Dispersive Spectroscopy) maps of silicon Ka1 line are displayed in Figure 3. EDS can be used as an indicator of coating coverage on the substrate surface. Elemental silicon is represented in blue; the continuity and relative intensity of the blue color reflect the continuity and relative thickness of the coating. A lack of blue signifies a discontinuity in the coating.
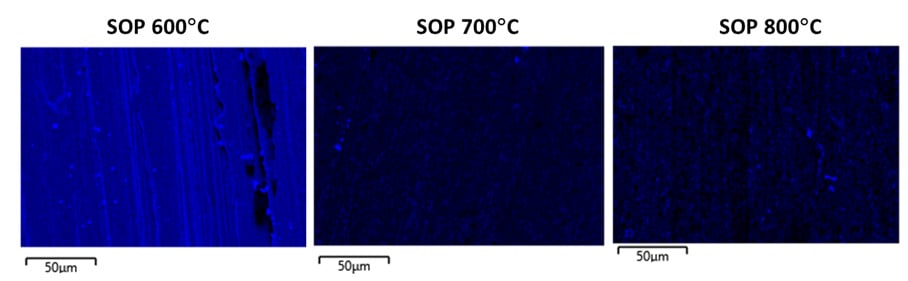
Figure 3: EDS maps of silicon Ka1 line (represented in blue), used as an indication for coating coverage, for Silcolloy coating after thermal exposure to 600, 700 and 800°C for 6 hours in air.
Figures 2 and 3 together deliver a consistent and clear message: the SOP coating suffers significant structural damage and coverage degradation as the temperature rises. At 600°C, the coating appeared smooth and unaltered under SEM, but small coating gaps started to show in the EDS map of SOP Silcolloy, likely due to the onset of crystallization.
At 700°C, the crystal grains broke out in islands, the silicon intensity became much weaker and the film coverage appeared mostly discontinuous for SOP. Finally at 800°C, the surface of the Silcolloy coating was dominated by a mixture of metal (from the 316L stainless-steel substrate) and crystalline silicon grains, and the silicon signal detected by EDS weakened even more.
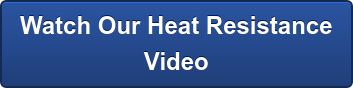
Summary
In this blog post we showed how Silcolloy® can change when exposed to very high temperatures. Exposure resulted in a different surface at 800°C compared to 600°C.
In our next blog post we'll discuss a new coating application method that maintains the surface characteristics, chemistry and corrosion resistance of Silcolloy but vastly improves the coating's performance when exposed to extreme heat. The next blog will discuss in detail the new coating application method and further testing results will be reviewed. The testing will show that the thermal performance of Silcolloy can be improved while the already excellent corrosion resistance and inertness properties of Silcolloy can be maintained.
To stay in touch with the latest in material research and coating performance, subscribe to our blog or follow us on LinkedIn.
